Explosion Control of Energy Storage Systems - Challenges + Opportunities
Stefan Kraft + Anil Kapahi
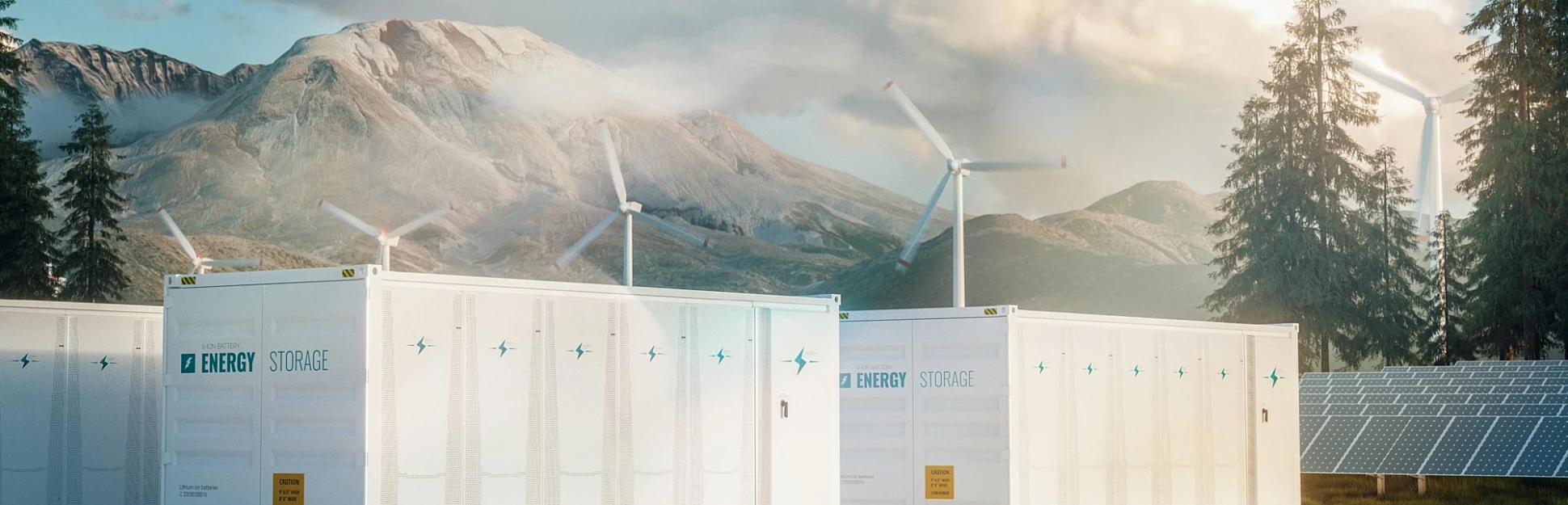
Nov 13, 2024
Introduction — ESS Explosion Hazards
Energy storage systems (ESS) are being installed in the United States and all over the world at an accelerating rate, and the majority of these installations use lithium-ion-based battery technology. For grid-scale and residential applications of ESS, explosion hazards are a significant concern due to the propensity of lithium-ion batteries to undergo thermal runaway, which causes a release of flammable gases composed of hydrogen, hydrocarbons (e.g. methane, ethylene, etc.), carbon monoxide, and carbon dioxide. The fire codes (IFC 2021 Chapter 1207, NFPA 855 ed. 2023) contain a requirement to include explosion protection for installed systems exceeding certain energy capacity thresholds. This requirement can be satisfied using passive protection methods such as deflagration venting according to NFPA 68 or active methods such as those specified in NFPA 69 (ed. 2024) that involve combustible concentration reduction using mechanical exhaust ventilation.
Both strategies have been used in the process safety industry for many years, but the design of modern ESS systems provides unique challenges with highly congested geometries in an enclosed environment and a stochastic hazard. Currently, technical gaps exist in the use of NFPA 68 and NFPA 69 for ESS containers, and thus a redundant approach is recommended to enhance safety. Although passive protection is a nearly maintenance-free solution, the prescriptive calculation methodology of a deflagration vent design according to NFPA 68 and its applicability to ESS-type designs is questionable. Active systems according to NFPA 69 such as mechanical exhaust ventilation are commonly used in the ESS industry, but their design is typically based on limited test data and an engineering analysis using assumptions for the progression of the failure event. Field incidents have different failure modes than those used in standardized battery testing such as UL 9540A, leading to ambiguous design bases. Furthermore, the need to perform routine inspections and the maintenance of backup power during a failure event reduces the reliability of active systems. The first part of this article highlights the applicability and limitations of active and passive strategies. In the second part, some specific gaps are identified in the NFPA 68 prescriptive deflagration vent area calculation methodology. A summary of available validation data is provided with a recommendation to create ESS-specific validation test data to fill existing gaps.
Current Protection Methodologies and Their Limitations
Economic factors in the energy storage industry typically lead to tightly packed ESS enclosures that cause difficulties in designing feasible explosion control solutions. Several competing design objectives for ESS can detrimentally affect fire and explosion safety, including the hot aisle/cold aisle layout for cooling efficiency, protection against water and dust ingress into the enclosure, and the use of larger cells with increased energy density. These objectives result in a highly congested enclosure with numerous obstructions, requiring only a small number of cells undergoing thermal runaway to create an explosible environment. Figure 1 shows the enclosure layout of the APS ESS installation that experienced an explosion event in 2019, which is an example of a congested enclosure using a large amount of equipment.[1]
The two types of explosion control options for ESS, NFPA 68 deflagration venting and NFPA 69 exhaust ventilation, are based on a design basis determined from UL 9540A test data. This testing is meant to provide baseline data for the analysis and is generally extrapolated to a sufficiently conservative hazard scenario for the ESS installation.
NFPA 68 systems require deflagration panels that yield at a pressure lower than the strength of the enclosure. This passive protection measure is designed to minimize damage by maintaining the enclosure’s structural integrity. The basis for the empirical models prescribed by NFPA 68 for calculating deflagration vent area are from experiments that are primarily relevant to the process safety industry and not ESS. For example, the experiments involved enclosure designs that do not resemble modern ESS enclosures with large battery racks. Typically, the hazards in these experiments involve a single flammable gas, unlike the mixture of flammable and non-flammable gases observed during a li-ion cell thermal runaway. In addition, the prescriptive methodology does not consider the dispersion of battery gas in the enclosure, and the resulting vent areas are based on homogeneous stoichiometric gas clouds. CFD models such as FLACS can address the dispersion of battery gas along with explosion analysis.[2] However, these models also lack a validation dataset directly applicable to ESS applications. Finally, this methodology requires deflagration to activate the panels, leading to battery gas accumulation if ignition does not occur.
NFPA 69 systems used in ESS are typically composed of a mechanical exhaust system that is activated upon detection of flammable gas to keep the global battery gas concentration below 25% of the lower flammability limit (LFL). The success of this approach depends on each aspect of the sequence of events operating as per design. If equipment maintenance is neglected, components may fail and lead to the exhaust ventilation system not activating during a failure event. Backup power is also a requirement for ensuring system availability during a failure event, but the duration can be difficult to define due to the stochastic nature of the failure. Designing an NFPA 69 system requires the release rate of battery gas, which is the most significant design input needed to size the exhaust fans. However, there is no standardized methodology to calculate this parameter, and it is not directly provided in the UL 9540A test report. A typical approach to determine this parameter is to perform an engineering analysis of UL 9540A test data. For most systems, the data available lacks statistical significance, and practitioners need to be cognizant of deficiencies in test methodologies to ensure the design basis is sufficiently conservative.
In general, using deflagration venting as passive explosion protection in addition to an active system has multiple benefits due to the nature of the battery failure event, which involves a rapid release of flammable gases. Exhaust ventilation normally depends on the detection of battery gas, but the accumulation of gas could cause a deflagration before the system fully activates. In addition, even with an active exhaust ventilation system, a thermal runaway event in an ESS can still result in a localized high concentration of flammable gas in certain zones that may be difficult to ventilate such as dead spots or stagnation areas. The aforementioned highlighted issues are further aggravated by rapid innovations in the ESS industry, which is witnessing a rapid increase in the energy density of individual cells. This higher energy density leads to a greater volume of flammable gas being released into the enclosure during a failure event. This makes it difficult to keep the global gas concentration below 25% LFL, particularly prior to exhaust fan activation. Thus, having a passive system (i.e., deflagration vent panels) to address the residual explosion hazard is beneficial by providing additional protection for the container.
Challenges in Passive Protection Methodology
The two main challenges in using passive protection methodology are design constraints for the enclosure and lack of validation data to support calculation methodology. These challenges make it difficult to obtain a feasible design for deflagration venting of ESS enclosures as the sole explosion protection scheme for most configurations.
The first challenge comes from the significant amount of equipment installed in the container and the limited area for installing deflagration vents. As mentioned, the battery racks often fill significant portions of the enclosure to economize space, but this reduces the free air volume. In addition, deflagration vents are installed on the ceiling to minimize potential exposures to surroundings and first responders. The amount of area for deflagration vents is typically limited to a portion of the ceiling.
When evaluating the feasibility of ESS enclosure deflagration venting designs, the flammability of the gas is normally the most important consideration as the actual physical design cannot be changed meaningfully for the pressure relief area calculation. The amount of hydrogen and hydrocarbons in the battery gas mixture has a significant influence on two parameters, laminar burning velocity (Su) and maximum explosion pressure (Pmax), which are directly correlated to the required deflagration vent area. The battery gas released during thermal runaway often has a large proportion of hydrogen, which possesses the most challenging explosion hazard.
A case study performed for a 20-foot (6.1-meter) ISO ESS container showed the difficulty of achieving a feasible design for a battery gas mixture that has a high laminar burning velocity (LBV).[3] A representative battery gas mixture (high LBV li-ion gas) of 43% hydrogen, 10% hydrocarbons, 37% carbon monoxide, and 10% carbon dioxide was used in the analysis. Henriksen reported burning velocity and maximum explosion pressure as 105.5 centimeters (41.5 inches)/second and 7.8 bar-g, respectively, for this mixture. [4] The NFPA 68 required vent area results for the high LBV li-ion gas are shown in Figure 2. The volume of battery gas at a stoichiometric concentration for a full-volume deflagration is 4143 liters (1,094.5 gallons), and the NFPA 68 required vent area for this scenario is over double the 8 square meters (86.1 square feet) available area on the ceiling of the container. Additionally, the partial volume calculations at stoichiometric concentrations also indicate a required vent area exceeding the available space. The exception is the 100-liter (0.3-gallon) volume, and for this amount, there is no required area, as the expected pressure rise is lower than the strength of the container. Note that the shaded bars indicate additional required area if the area of obstructions is twice that of the modeled value. Due to the different types and configurations of obstructions in ESS containers (e.g., conduit, busbars, sprinkler or other fire protection equipment, battery racks), the value used in the NFPA 68 calculation will vary from design to design. For ESS enclosures, as demonstrated by the above example, battery gas mixtures that have greater than 30% hydrogen are difficult to protect with deflagration venting as the sole explosion protection measure due to the high laminar burning velocity. It is common for the gas released during thermal runaway to contain more than 30% of hydrogen, but for some mixtures that have a corresponding high level of carbon dioxide, the flammability properties are reduced to a level at which it is feasible to use deflagration venting as the explosion control solution.[3]
The second challenge is related to calculations within NFPA 68 that are not validated for ESS enclosures and associated explosion hazards involving battery gas. NFPA 68 has been developed by the technical committee based on a substantial amount of experimental data primarily relevant to the oil and gas industry. Periodically, its calculation approach has been modified to account for different aspects of explosion phenomena. For example, the 2013 edition added an approach to account for the effects of internal obstructions causing turbulence of the flame front. The data used as the basis for this update was surveyed by Zalosh, and most of the experiments had equipment such as piping arrays, which are relevant to the process safety industry (e.g., petrochemical).[5] These obstructions are different compared to the large battery rack units and electrical equipment typical in the energy storage industry. In addition, the flammable gas hazard is different in that it is a mixture of hydrogen, carbon monoxide, hydrocarbons, and carbon dioxide rather than a single flammable gas species. Considering all these factors, the NFPA 68 deflagration venting method needs to be evaluated by the industry to determine if the underlying assumptions for characterizing the hazard and physical phenomena involved are still applicable.
Gaps in Validation Dataset and Calculation Methodology
Although a test program on ESS container deflagration vent area assessment is not available in the public literature, the HYSEA project conducted a series of tests with hydrogen released in 20-foot (6.1-meter) ISO containers that are to some degree relevant to ESS container explosion mitigation.[6] The enclosures used in the HYSEA testing were standard ISO 20-foot (6.1-meter) containers with a modified roof to enable testing with 4-, 6-, and 8-square-meter (41.1-, 64.6-, and 86.1-square-foot) areas of deflagration vent panels. The container dimensions as tested were the same as for ESS containers, but the internal layout is different, as most tests had few or no obstructions. The obstructions that were present did not represent battery racks. However, the test program’s use of various hydrogen concentrations and a range of ceiling deflagration vent areas give results that are useful in evaluating the performance of NFPA 68 for an ISO-style container for this highly flammable gas. In addition, the work is beneficial in designing experiments relevant to ESS explosion research. An example of one of the tests is shown in Figure 3, in which 6 total 1-meter x 1-meter (39.4-inch x 39.4-inch) vent panels opened upon deflagration of a stratified mixture of 21% hydrogen gas. The test resulted in a maximum overpressure of 0.611 bar-g and significant permanent deformation of the container. Typically, in experiments for which overpressure was limited to the range of 0.1 to 0.3 bar-g, deformation of the container was less than 0.2 meters (8 inches), and no catastrophic failure resulted.
The default method used in NFPA 68 calculations is to use the maximum burning velocity and explosion pressure for the full volume calculations, which for hydrogen is 286 centimeters (112.6 inches)/second and 6.8 bar-g. However, it was noted in NFPA 68 Annex K “Example Vent Sizing for Hydrogen Mixtures” that a burning velocity and explosion pressure at a different concentration (e.g., lean mixture) is appropriate for use in vent sizing if justified by the hazard analysis.[7] To evaluate the HYSEA test data that is most relevant to the ESS industry, this approach was used for the range of hydrogen concentrations. Experimental deflagration pressures from the tests are shown in Figure 4 along with predictions of pressure from NFPA 68 using the burning velocity and explosion pressure for the specific concentration of hydrogen.[6] Data and predictions are presented for containers with 6 square meters (64.6 square feet) of deflagration vent area. The experimental data includes tests that had very few obstructions as well as tests that had a pipe rack in the center of the container. In general, the predictions of pressure using NFPA 68 are conservative except for the high congestion tests with low amounts of hydrogen and tests using stratified mixtures. Although the overall concentration in the container was low (18-21%) for the stratified mixture tests, the local hydrogen concentration was greater. These results illustrate that although the methodology is sound for containers with homogenous fuel-air mixtures and few obstructions, additional research is needed to broaden its applicability. ESS containers have significant congestion and dispersion of lithium-ion battery gas could result in stratification within the enclosure. Therefore, a dataset specific to ESS applications would help in validating the efficacy of both the NFPA 68 methodology and other calculation approaches such as CFD modeling.
The default calculation methodology within NFPA 68 assumes a homogeneous stoichiometric mixture. Although NFPA 68 permits using lean mixture properties (burning velocity and explosion pressure) for determining partial volume requirements for deflagration vent area, the default method is to evaluate the area for the full volume (stoichiometric or slightly rich) properties and calculate a reduction factor based on the empirical partial volume correlation given in the standard. This approach is more conservative than using the lean mixture flammability properties with the full volume equation approach. A comparison of the two approaches was done using a representative ESS container geometry with battery racks as obstructions, using the same configuration and parameters as in the previously mentioned feasibility study.[3] Lithium-ion battery thermal runaway gas flammability properties were obtained from Barowy et al.[8] The results are shown in Figure 5. The volume of battery gas in the container is shown on the upper x axis, while the global concentration corresponding to that volume is on the lower x axis.
The required vent area for the full volume deflagration case is 7.2 square meters (77.5 square feet). The deflagration vent area requirement of the two methods is compared with respect to battery gas concentration. The default method, using the empirical model given in NFPA 68, has a requirement of higher vent areas compared to the well-mixed lean mixtures calculation method. For a global concentration corresponding to 25% LFL, which is a safer threshold according to NFPA 69, the required area is 3.9 square meters (42 square feet) using the default methodology. Note that for this volume the overall concentration is only 2.6%. For the lean mixtures, the required deflagration vent area is 0 square meters at or below the LFL (10.5% battery gas concentration.) A realistic failure scenario could evolve into a heterogenous cloud, and test data corresponding to bounding cases will be useful for practitioners to define a credible conservative scenario and how it relates to predictions using the two methods. In addition, test data corresponding to stratified mixtures with a gradient in concentration could also provide useful insights similar to HYSEA experiments.
Summary and Opportunities
Due to the stochastic nature of the lithium-ion battery explosion hazard and the lack of validation data specific to ESS application, redundancy in the explosion control approach is recommended. NFPA 68 and 69 have value for protecting life and property and should be used in conjunction with one another. UL 9540A data is typically used to establish the hazard of flammable gas in ESS containers for explosion mitigation modeling. However, there is no systematic approach accepted by the industry to define the design basis using this data, and the test itself does not bind all failure modes. Thus, even with prescriptive code requirements such as NFPA 68, the hazard itself is based on an engineering assessment that requires a vast understanding of battery failure. There are also a significant number of assumptions required to complete the analysis, most of which cannot be verified using the available test data.
NFPA 68 predictions of overpressure during vented deflagrations are conservative for some configurations that are similar to ESS enclosures demonstrated by the HYSEA tests for 20-foot (6.1-meter) ISO containers. However, these tests did not include large obstructions and narrow aisles (from battery racks), which are typical in ESS. Thus, a dedicated experimental test program is required to create a validation dataset that can be used to evaluate the applicability of the current calculation methodologies for this type of geometry. In addition, the battery gas flammability characteristics at different concentrations are required to perform the dispersion analysis to justify the usage of explosible cloud concentrations other than stoichiometric. These gaps offer a significant opportunity for the fire protection community to create a publicly available validation dataset relevant to ESS enclosures.
References
[1] M. B. McKinnon, S. DeCrane, and S. Kerber, 2020, Four Firefighters Injured in Lithium-Ion Battery Energy Storage System Explosion — Arizona, Underwriters Laboratories, Columbia, MD.
[2] Gexcon AS, 2021, FLACS-CFD V21.3 User’s Manual.
[3] S. Kraft, S. and A. Kapahi, 2024, “Applicability of NFPA 68 for ESS Enclosures,” Proceedings of the 15th International Symposium on Hazards, Prevention, and Mitigation of Industrial Explosions (ISHPMIE), 2024, Naples, Italy, pp. 596–611..
[4] M. Henriksen, K. Vaagsaether, J. Lundberg, S. Forseth, and D. Bjerketvedt, 2021, “Laminar Burning Velocity of Gases Vented from Failed Li-Ion Batteries,” J. Power Sources, 506, p. 230141.
[5] R. Zalosh, 2008, Explosion Venting Data and Modeling Literature Review, Fire Protection Research Foundation, Quincy, MA.
[6] T. Skjold, M. Lucas, H. Hisken, G. Atanga, S. Lakshmipathy, L. Bernard, M. van Wingerden, K. van Wingerden, J. X. Wen, V. C. M. Rao, A. Sinha, M. Carcassi, M. Schiavetti, T. Pini, J. Snoeys, A. G. Hanssen, C. Wang, S. Jallais, E. Vyazmina, D. Miller, and C. R. Bauwens, 2020, “Review of the HySEA Project,” ISHPMIE 2020, Braunschweig, Germany.
[7] NFPA, 2023, NFPA 68: Standard on Explosion Protection by Deflagration Venting 2023 Ed., NFPA, Quincy, MA.
[8] A. Barowy, A. Schraiber, and R. Zalosh, 2022, “Explosion Protection for Prompt and Delayed Deflagrations in Containerized Lithium-Ion Battery Energy Storage Systems,” J. Loss Prev. Process Ind., 80, p. 104893.
Republished with permission from SFPE. Original article can be found here.
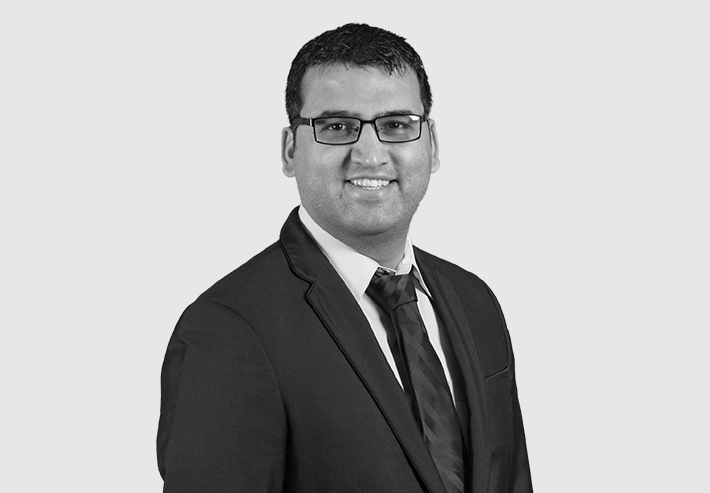
About the author
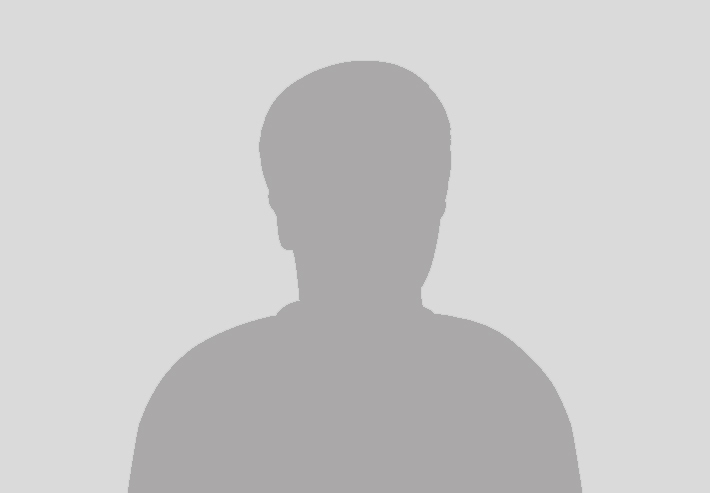
About the author